|
|
Research Topics |
 |
|
|
Nanoscale corona discharges in liquids for optical emission spectroscopy |
Light emitted from a new form of cold plasma in liquid – field emission generated, highly non-equilibrium and high energy density – permits Optical Emission Spectroscopy (OES) analysis of the elemental composition of solutions within nanoseconds from femtoliter volumes. The technology is being investigated as an elemental detector comparable to laser induced breakdown spectroscopy (LIBS) in liquids only smaller and as a optical pump for fluoroprobes. The research was featured in the journal Nature as a News and Views article Analytical chemistry: Plasma bubbles detect elements. There they highlighted the probe's small size, novel plasma, and potential impact on analytical chemistry. The paper has also received coverage on two science news web sites, nanotechweb.org and nanowerk.com. |
|
|
 |
|
Discharges in liquids for lightening of heavy oils |
With dwindling world oil reserves, non-traditional heavy oils are a potential sources for future petroleum based energy needs. Heavy oils however are difficult to extract, transport and refine. The discharge under investigation in this research relates to hydrotreating and upgrading of heavy crude oil containing water by subjecting the oil to microscopic electrical discharges generated between water droplets or other charged particles in the oil. When subject to an electric field charged water droplets in the dielectric oil deform and propagate through the oil partially bridging the discharge gap and locally increasing the electric field sufficiently to initiate plasma discharges through the oil. Taylor cones formed by the water droplets can be microscale and are sufficiently sharp to penetrate even the most viscous oils. The nature of the microscopic discharges is similar to that of the nanoscale discharges in liquids. The chemistry at the the oil-water-plasma interfaces can promote upgrading of the heavy crude oil. View a video of the particle and discharge dynamics. |
|
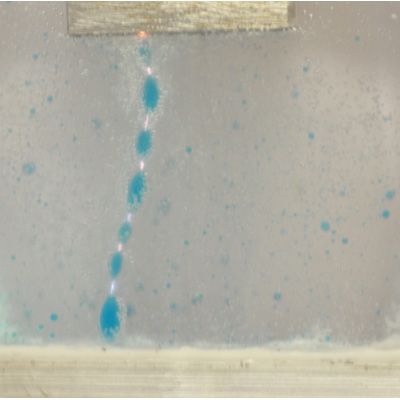 |
Discharge between conducting water droplets in mineral oil. |
|
|
 |
|
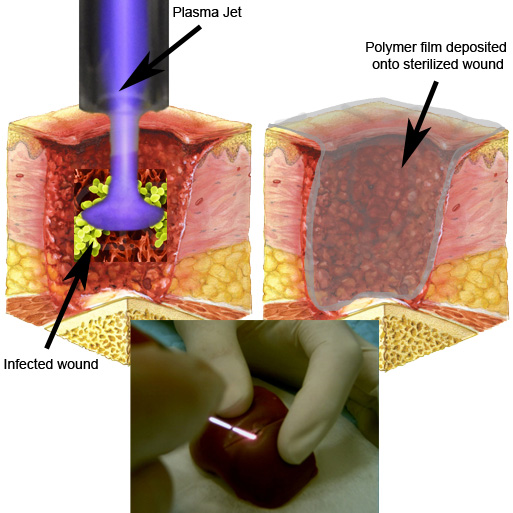 |
Schematic of plasma enhanced chemical vapor deposotion (PECVD) process for sterilization and sealing of wounds |
|
Plasma enhanced polymer film deposition onto , biological and living substrates at ambient conditions |
Why would we want to do ‘PECVD on people’? In medical procedures living tissue interact with manufactured bio-materials to various degrees of complexity, whether it is: electrode arrays in peripheral nerves for connections to prosthetics, artificial hip implants, inserted stents, or simple bandages. The development of ‘PECVD on people’ techniques allows for manufacturing of the materials in-situ and in-vivo. For example: the bonding of artificial implants to bone, in-vivo synthesis of metallic, dielectric, or polymeric implants, in-vivo repair of implants, or the deposition of bandages or adhesive sutures. The advantage of PECVD over simpler techniques such as cements and prefabrication is case specific. PECVD allows for a high level of control, great adhesion, and unique materials and processes. A potential application is the deposition of super sterile multifunctional bandages as illustrated in Figure 1. In this illustration an infected wound (like a burn or ulcer) is sterilized and coated with a polymeric film. The thin film would be conformal to the wound and could prevent further infection. By controlling parameters of the deposition the film could have functional or spatial variations, such as: cell growth promoters or antimicrobial compounds doped into the film, porosity, or biodegradability. This bandage deposition process could build upon the plasma’s unique sterilization capabilities, combining the sterilization and deposition steps. For more information view our poster or see a video on plasma jets. |
|
|
 |
|
Direct write surface patterning using micro and nano-scale plasma |
Traditional techniques of patterning small features (100 nm to 10 um) onto surfaces, whether for integrated circuit (IC) manufacturing or for micro-electromechanical system (MEMS), has relied on the use of photolithography and masking to attain the high resolutions. After masking the surface is exposed to a plasma etching or deposition process. Though very robust and highly parallel in some applications drawbacks of such techniques are the multistep nature of the process and the static nature of the processing. Utilizing the inherently small size of microplasmas and nanoplasmas small features and patterns can be created on surfaces directly without masking. The small plasmas can create very localized change. The quality and resolution of this
technique for surface patterning depends on our ability to create a controllable small scale plasma discharge which can perform localized deposition. Research in this area focuses on the stability and uniformity of the discharges, scaling of gas a liquid phase discharges to sub-micron levels, and maintaining appropriate non-thermal plasma conditions |
|
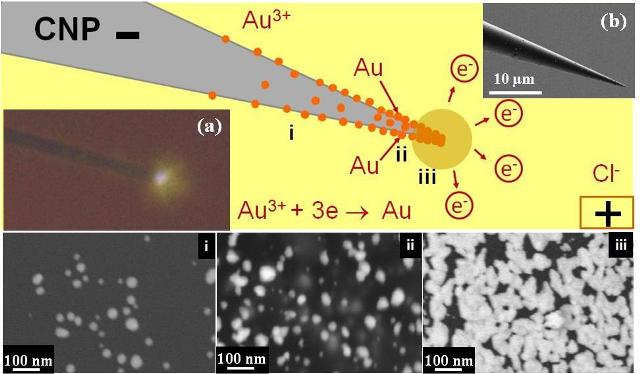 |
Schematic diagram showing the mechanism of gold nanoparticle formation and deposition at the tip of a carbon nanotipped probe. Panels i-iii show the concentration gradient from the tip. Insets: (a) Optical micrograph of a typical corona in a AuCl4.xH2O solution, and (b) CNP tip with deposited Au NPs |
|
|
 |
|
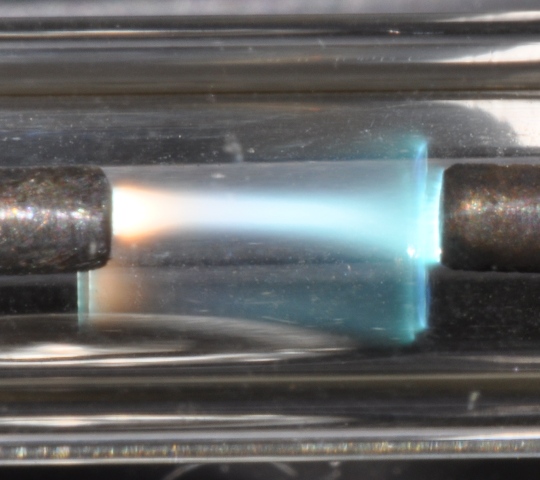
Discharge in methane reformer |
Efficient Plasma discharge reforming of methane |
Reforming is a process in which abundant fossil fuels are converted into other useful hydrocarbons and fuels. A common process is the conversion of natural gas (primarily methane (CH4)) into hydrogen (H2), ethylene (C2H4) and acetylene (C2H2). Hydrogen has applications in fuel cells, ethylene is the precursor for creating polyethylene plastics, and acetylene is also a fuel and chemical building block. Reforming occurs at enormous industrial scales. Smaller scale reforming is also of interest for vehicle based fuel cells and other on demand fuel conversions. Most essential in these application is efficient processing useful and cost effective. Common large scale processing uses high pressure thermal cracking to breakup and react the hydrocarbons in a thermal process. In small scale reactor these high temperature lead to inefficiencies. Non-thermal plasma discharges can be used for more efficient reforming. The energy input into the plasma and the electrons couple with the vibrational modes of the molecules. In a non-thermal plasma the molecules have more vibrational energy than is to be expected based upon their temperature. This vibrational energy can accelerate chemical reactions (especially endothermic reactions) without the need for high temperatures. This research attempts to carefully qualify this efficiency enhancement based upon measurable quantities such as the gas temperature, vibrational temperature, and residence time of the molecules in the plasma |
|
|
 |
|
Swithcing and stability of high pressure non-thermal plasmas |
Stable direct current plasmas at pressures up to 10 atmospheres can regularly be generated in air using microplasma systems. Rapid cooling due to the small size is typically suggested as the thermally stabilizing mechanism. However, temperatures of the stable discharges are significantly higher than ambient, and stable operation is not easily achieved in all gases at similar sizes. Analyzing of the thermal instability, we find that the inclusion of the simple ballasted external circuit in the analysis leads to additional stabilizing mechanisms. This stabilization occurs in microplasmas due to the characteristic times of the external circuit and the instability being comparable, which allows the electric field to change during the time frame of the instability. Experimentally this is implemented by reducing the stray capacitance of the external circuit. We are investigating the stability of microplasmas in several gases and at high pressures. In applications we are using the understanding of the stability and instabilities associated with these discharge to create microscale plasma transitions |
|
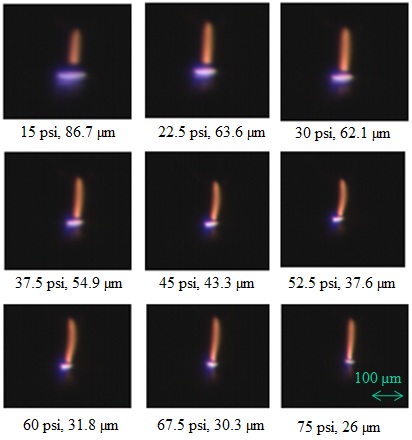
Scaling of Nitrogen glow discahrges at high pressure |
|
 |
|
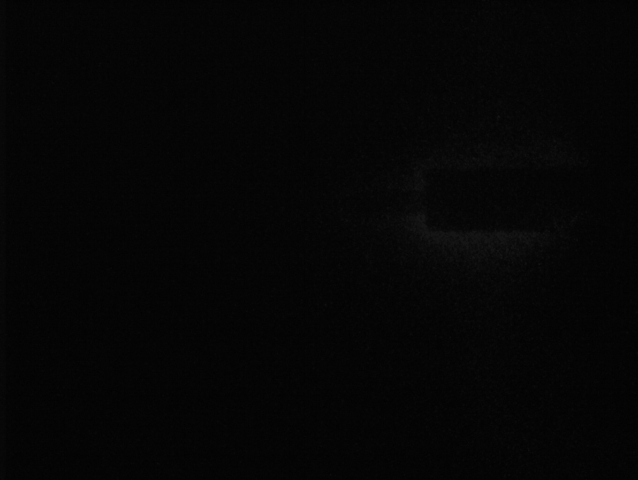 |
Initial tests of 1.5 mm DPF |
|
High efficiency microscale dense plasma focus neutron source |
A highly efficient neutron source at high deuterium/tritium pressures will be achieved by miniaturization of the dense plasma focus (DPF) plasma device from typical cm sizes to micron scale with increasing pressures (0.01 atm to 10's atm). The DPF efficiency should scales as Q~ P^4*a (P=pressure, a = device radius). Challenges will be maintaining discharge breakdown, driving, and pinch scaling parameters and generating sub-ns rise time voltage pulses. Applications for the small high efficiency neutron source could be a portable neutron based improvised explosive device (IED) detector, or for the neutron trigger for starting small subcritical nuclear assemblies to be used on board spacecraft for electric propulsion.
In collaboration with [FT]^2L research by Andrew Duggleby |
|
|
 |
Microplasma Actuators |
This research relates to a novel flow control methodology for airfoils undergoing dynamic stall.
Texas A&M University along with Lynntech, its STTR partner is developing novel pulsed plasma discharge based actuators for flow control on dynamically stalled airfoils. The technology consists of pulsed micro-spark plasma actuators which will induce high velocity airflow within the airfoil boundary layer, thus reattaching the flow. The proposed plasma actuator should be able to achieve high Reynolds number (>5 million) flow control compared to contemporary dielectric barrier discharge plasma actuators. Advantages of the system include low power consumption, ease of installation, increased flight stability, reduced drag and higher stall angles.
|
|
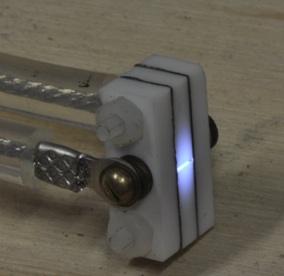
Pulsed microplasma discharge actuator. |
|
|
|
|
 |
|
|